Volcanoes: Terror From Below
By Brian Rohrig | February 2021
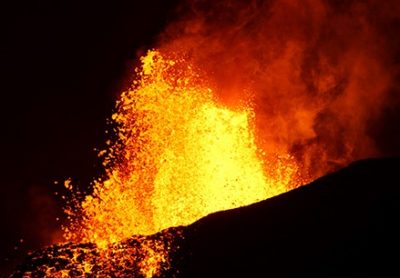
Few things in nature can match the raw power of a volcanic eruption. 1816 is famously known as the “year without a summer” due to the eruption of Mount Tambora in Indonesia. Its eruption the previous year sent prodigious amounts of sulfur dioxide (SO2) into the atmosphere, which stimulated cloud formation and triggered global cooling. In 1883, another Indonesian volcano, Krakatoa, erupted with such force that it could be heard 4,800 km (3,000 miles) away. That would be like setting off a fireworks display in Boston that could be heard in Los Angeles.
In a much more recent reminder of volcanoes’ might, Kilauea on Hawaii’s Big Island erupted explosively in 2018, shooting lava 90 meters (300 feet) into the air in a dangerous fountain as tall as the Statue of Liberty. Rivers of lava spilled down the side of the volcano and destroyed hundreds of homes. When lava entered Hawaii’s largest freshwater lake, the water completely evaporated in a matter of hours. In December 2020, Kilauea erupted again.
Krakatoa’s 1883 eruption was one of the deadliest in modern history. It’s estimated that more than 36,000 people died due to the event. Improved monitoring and understanding of volcanoes, however, have saved thousands of lives in more recent years.
The terrifying mystery of how volcanoes form is made less so by examining the earth right under our feet.
What is Earth made of?
On a normal day for most people, volcanic eruptions are not at the forefront of daily life. Earth’s outer layer, or lithosphere, consists of the solid crust and brittle upper portion of the mantle. It is reassuringly stable—for the most part. Below the lithosphere is the asthenosphere, a ductile layer of the upper mantle.
Below the asthenosphere sits the rest of the mantle, and under that is the dense core, which is thought to be composed mostly of iron and nickel. As far as we know, the outer core is mostly composed of liquid iron and nickel. The inner core is largely solid iron.
So, you might assume that magma originates in the outer core—it’s already liquid. But magma’s chemical composition tells a different story. It’s made primarily of molten silicate rocks.
Silicates are minerals built from base units containing silicon atoms bonded to four oxygen atoms, which are then built up in different ways. These silicate rings and sheets carry negative charges, which allows positively charged ions to combine with the silica tetrahedra to form minerals. This is the type of rock found in the solid mantle. This clue about where magma comes from raises the question: How does an otherwise solid layer of silicate rock liquefy?
If there’s one thing we’ve learned about turning solids into liquids, it’s that adding heat can do the trick. And in everyday circumstances that we observe, say in a classroom lab, heating a solid, such as ice, will cause it to melt. The circumstances deep inside our planet, however, are not what most of us think of as normal.
Mantle temperature estimates range between 1,000 °C in the upper mantle near the crust and 4,000 °C close to the core. Temperatures above 1,500 °C are hot enough to melt mantle rock, but there’s a catch. The intense pressure within the mantle prevents the molecules from entering a free-flowing liquid state. Instead, they settle into a quasi-solid, ductile phase, which means the rocks can flow in response to deformation like Silly Putty, though obviously they’re much harder.
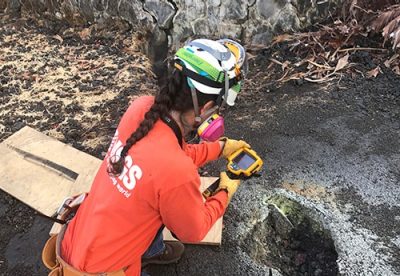
Because the lower mantle is hotter than the upper mantle, convection occurs. Convection
happens when a material heats up, becoming less dense, and rises through another substance. But as it rises, it starts to cool and become more dense. Then it sinks. This same phenomenon is what causes liquid blobs in a lava lamp to rise and fall.
Unlike a lava lamp, however, convection in the mantle occurs at a glacial pace—geologists estimate material in the mantle only budges a few centimeters per year. Still, the convection of the mantle is believed to be responsible for breaking up the lithosphere (the crust and the upper part of the mantle) into large plates.
It’s important to understand this slow dynamic because all sorts of trouble arises at the boundaries where plates meet. It’s also what contributes to the formation of magma.
The making of magma
So far, we still haven’t seen how rock in the mantle can melt into magma. Research on magma’s chemical composition and other data suggest that magma forms in the upper mantle at a maximum depth of about 200 km, where temperatures can reach up to 1,800 °C. At this depth, however, the solidus of peridotite, the dominant type of rock in the upper mantle, is 2,000 °C. The solidus is the temperature at which rock starts to melt.
So, how does magma form? There are at least a couple of conditions that can change to cause rock to melt in the upper mantle. Let’s take a look at a couple of scenarios to see how this is possible.
In the first scenario, imagine two of Earth’s tectonic plates slowly moving apart. This divergence decreases pressure on the underlying rock, lowering the rock’s melting point. Pressure on rock also decreases as it moves upward through the mantle on a convection current. When solids melt due to a decrease in pressure, this is called decompression melting.
Under a different scenario, when tectonic plates converge instead of drift apart, rocks will melt due to another condition change. One plate slips below another in a process known as subduction. As the cool crust of one plate is driven downward to the hotter temperatures of the upper mantle, water is released from hydrous minerals, such as serpentine, which is 13% water by weight. Introducing this water to the mantle creates an interesting effect—it lowers the melting temperature of the rock. As an impurity, water added to a crystal at high temperature and pressure lowers its melting point. The polar nature of water causes bonds to weaken in the solid rock, making it less rigid. Since the rock is very hot, there is plenty of available heat to melt it, turning rock into freely flowing liquid rock. This process is called flux melting.
Will it explode?
Now that we have melted rock, what’s next?
As magma moves upward toward the surface (remember our lava lamp comparison), the pressure on the melted rock decreases. Less pressure allows dissolved gases within the magma to expand, similar to how bubbles form when you uncap a bottle of soda. As gases expand within the magma, it becomes even less dense and thus more buoyant, hastening its rise to the surface.
Carbon dioxide (CO2), and sulfur dioxide (SO2), which has a strong irritating odor, like that of a lit match. Hydrogen sulfide (H2S), which has a characteristic rotten egg smell, is often produced in smaller quantities.
For a volcano to form, however, there must be some type of fissure or break in the surface of the Earth’s crust to let the magma and gases out, which is why you typically see volcanoes at plate boundaries. Once the magma breaks the surface, it is called lava.
Gases can play an important role in a volcano’s eruption. If gas pressure builds up within the magma, it can result in an explosion. This happens when magma is highly viscous.
The viscosity of magma depends heavily on a couple of factors: temperature and composition. Cooler temperatures and greater silicon dioxide (SiO2) content make magma more viscous. SiO2, also called silica, forms a strong crystalline structure known as a network covalent solid, in which silica molecules covalently bond to each other. Gases have a hard time escaping from this type of structure.
Explosive eruptions are more likely to eject solid matter, known as tephra, into the air, which can range from fine ash to giant boulders. Pumice—porous, volcanic rock that floats—is formed during an explosive eruption. Holes are created when gases escape from superheated rock and leave pores as the rock quickly cools, like the holes formed when CO2 gas escapes from a loaf of bread rising in the oven.
If large amounts of solid material are ejected rapidly, a pyroclastic flow can result, in which the solid material, mixed with hot gases, moves like an avalanche down the side of the volcano. These flows can reach speeds of more than 80 km/h (50 mph), obliterating everything in its path. Many more deaths have occurred from pyroclastic flows than lava flows. The 57 deaths from the Mt. St. Helens eruption in Washington in 1980—the deadliest volcanic eruption in U.S. history—were primarily due to asphyxiation from the pyroclastic flow of ash.
The Kilauea volcano in Hawaii has erupted both explosively and nonexplosively in the past, recently entering a nonexplosive, or effusive, phase. Effusive eruptions are characterized by continual lava flows, which can be quite spectacular to witness.
At one point, lava was pouring out at a rate of nearly 100,000 liters per second. Its top speed was reported to be around 27 km/h (17 mph), which doesn’t sound very fast, but unless you’re Olympic athlete Usain Bolt, you wouldn’t be able to outrun it.
If you look up photos from Kilauea’s 2018 eruption or remember them, you’ll pull up striking images of fiery lava exploding from the volcano crater and flowing down its sides. You can roughly estimate the lava’s temperature from its color, owing to incandescence, which is the emission of visible light from hot objects.
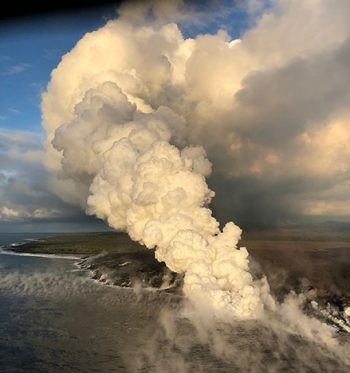
Any object will emit visible light if heated to a high enough temperature. The hottest lava is yellow, at around 1,000 to 1,200 °C. The lava that boiled away Hawaii’s Green Lake was a toasty 1,170 °C. Orange is cooler, while red is the coolest, coming in at 600 to 800 °C.
Deadly heat isn’t a volcano’s only dangerous product. As lava from Kilauea made its way to the ocean, it created yet another hazard, producing a toxic fog nicknamed laze, short for lava haze. The caustic haze can cause difficulty breathing and irritate the eyes and skin.
Laze forms when super hot lava pours into the seawater, boiling it to dryness, producing steam and fine bits of volcanic glass. Particles of solid salts also form. The salts are primarily NaCl, but the less abundant MgCl2 reacts readily with the hot steam to form HCl.
MgCI2(s) + H2O(g) → MgO(s) + 2 HCI(g)
Today, because we have a much better understanding of the science behind eruptions, scientists can predict when they will happen by monitoring earthquakes and volcanic gas releases. Residents near volcanoes can now often be evacuated before an eruption occurs. Forecasting can’t save homes, but it has saved tens of thousands of lives.
Volcanic eruptions are not as rare as you might think, with about 20 eruptions occurring on any given day. In all likelihood, at this very moment a volcano is erupting somewhere in the world.
REFERENCES
Ruth, C. Letting Off Steam. ChemMatters, April 2009:
http://wychem.scienceontheweb.net/ChemCD/ChemMatters/2009_4/letting_off_steam.pdf [accessed Dec 2020].
Consensus Study Report. Volcanic Eruptions and Their Repose, Unrest, Precursors, and Timing. The National Academies Press: Washington, D.C., 2017: https://www.nap.edu/catalog/24650/volcanic-eruptions-and-their-repose-unrest-precursors-and-timing [accessed Dec 2020].
Nelson, S. A. Tulane University. Volcanoes, Magma, and Volcanic Eruptions, Sept 14, 2015: http://www.tulane.edu/~sanelson/Natural_Disasters/volcan&magma.htm [accessed Dec 2020].
Answer to the question on p. 11: 1.55 x 1014 J
Brian Rohrig is a chemistry teacher based in Columbus, Ohio.
How an Entire Lake Vanished
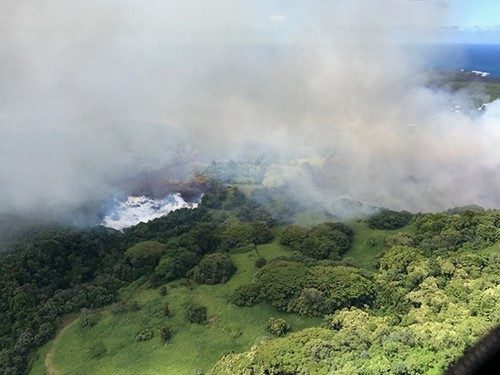
In a stunning demonstration of how volcanoes can dramatically alter landscapes, Kilauea’s 2018 eruption boiled away Hawaii’s biggest freshwater lake. See if you can figure out how much energy it took to empty the basin of all its water.
To come up with an estimate, let’s make the following assumptions: 1) the lake is initially full and is hemispheric in shape; and 2) its deepest point is 30.5 meters (about 100 feet) below the surface. That gives us a water volume of about 59,400 m3. Now let’s also assume that the lake’s starting temperature is 18°C (64°F), and that the lake water has the same density, specific heat capacity (4.184 J/g°C) and heat of vaporization (40.6 kJ/mol) as pure water. How much energy did it take to boil off all the water in the lake?
Also in this Issue...
Open for Discussion:
Deciding What's Right
Science isn’t just about clear-cut facts and figures. Sometimes, it calls for deciding right and wrong.
By Michael Tinnesand

Ethical dilemmas are some of the most difficult problems we have to deal with in life. Acting ethically involves deciding what’s right based on several considerations, including honesty, integrity, fairness, and keeping others’ interests in mind.