The Ozone Layer: Our Global Sunscreen
By Mike Carlowicz April 2013
It's not often that scientists get to conduct experiments that seem like they come out of a science fiction novel or a video game. Yet, that is what some researchers at NASA did a few years ago.
Atmospheric physicists Paul Newman and Luke Oman built a simulation of the Earth’s atmosphere and then proceeded to strip away our protective ozone layer. Their computer model reproduced the chemistry and circulation of the air; the natural variations in temperatures and winds; and the minor changes in the amount of energy received from the sun. Newman and Oman then added ozone-destroying chemicals to the atmosphere at a rate of 3% more per year—on top of what was already in our 1970s atmosphere. For several months, they ran their model on a supercomputer and reproduced about 80 years of simulated Earth time. They called their experiment "The World Avoided."
By the year 2020 in the simulation, 17% of the Earth's protective ozone layer vanished. Holes in the ozone layer formed not just over Antarctica—as they currently do each spring—but over the Arctic, too. By 2040, the ultraviolet (UV) index, the measure of the sunburn-causing radiation reaching the Earth's surface, rose as high as 15 on summer days in mid-latitude cities such as Washington, D.C. (A UV index of 10 is considered very high today and quickly leads to sunburn if you don't wear sunscreen.)
In the simulated future, two-thirds of the planet's ozone layer disappeared by 2065. Ozone holes swirled over both poles all year long, and most ozone disappeared from the tropics, too. The intensity of UV radiation reaching the Earth's surface doubled—levels that would increase DNA mutations in human and animal cells, suppress our immune systems, and increase the incidence of cataracts and skin cancer.
In another demonstration of the effects of a world without an ozone layer, Newman's group exposed a basil plant to intense UV radiation. In a lab at NASA's Goddard Space Flight Center, they set up a special lamp that simulates the intensity of sunlight when testing satellite hardware. They put a basil plant in front of that lamp and a camera behind it and watched for 27 hours. Without the protective shielding of an ozone layer, the plant started browning and developing spots within an hour. It was dead in a day.
“We simulated a world avoided, and it’s a world we should be glad we avoided,” Newman said.
A good idea with a bad outcome
The experiments run by Newman and colleagues were actually extensions of an experiment that humans have been unwittingly running with the Earth for nearly a century. Humans have been depleting the ozone layer with chemical products.
The unintentional experiment started in the late 1920s, when Thomas Midgley and other industrial chemists began to produce chlorofluorocarbons (CFCs), nontoxic compounds that improved refrigeration. Other manufacturers later put these chemicals to work as propellants in spray cans and as solvents for cleaning greasy residues. CFCs and similar compounds are mostly inert (nonreactive) at sea level and so were found to be extremely useful for all of these tasks.
But the progress came with a cost: People who used CFC products were releasing more chlorine into the environment than could be removed by natural processes. Unknown to the chemists who developed them, CFCs were accumulating and dispersing through the atmosphere. At high altitudes, where conditions are different from the Earth’s surface, those chlorine compounds were destroying ozone, the gas that absorbs and scatters UV light from the sun.
Because CFCs are so stable near the ground, it took decades before scientists started seeing signs of this ozone problem higher in the atmosphere. It wasn't until the 1970s that chemists learned that CFCs break into chlorine atoms when exposed to intense UV light from the sun. A chlorine atom can react with ozone to form an oxygen molecule (O2) and a molecule of chlorine monoxide (ClO), and the chlorine atom is “regenerated” when the chlorine monoxide molecule reacts with another ozone molecule. The chemical reactions are as follows:
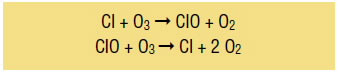
The net result (2O3 à 3O2) is that one chlorine atom destroys two ozone molecules and is regenerated in the process, allowing it to react over and over again. So, a single chlorine atom can destroy thousands of ozone molecules. And because CFCs are removed very slowly from the atmosphere, their ozone-depleting power persists for decades after they are emitted.
As a result of the widespread use of CFCs in consumer products, the ozone layer became depleted, particularly through a phenomenon that most of us know as "the ozone hole." The word "hole" should not be taken literally; there is no hole in the sky. But the area has significantly less—as much as two-thirds less—protective ozone to shield the Earth's surface.
In the past decade, ozone holes have stretched as much as 29 million square kilometers across the skies over Antarctica. Residents of New Zealand and Australia are warned each year to take precautions from the sun, as the ozone hole can occasionally stretch from the South Pole toward middle latitudes.
How Do You Find and Study a Hole in the Atmosphere?
Atmospheric ozone can be measured both directly and remotely. Scientists make direct measurements by launching instruments on balloons or by carrying them up on research airplanes. The instruments use chemical reactions or pass UV light through a sample of air to measure the presence and amount of ozone.
Remote measurements of ozone are made by instruments on the ground and on satellites; in either case, the instrument of choice is usually a spectrometer or photometer—a device that measures how gases absorb or emit light. In the 1920s and 1930s, British scientist Gordon Dobson first used photographic plates and then a spectrophotometer—a device that consists of a spectrometer and a photometer—to measure differences in ultraviolet light wavelengths reaching the Earth’s surface. Although the instruments have been modernized, the basic premise of Dobson's work continues to this day. The most common unit of measurement for atmospheric ozone levels—known as the Dobson unit (DU)—was named in his honor.
Scientists first became aware of the depletion of the ozone layer in 1985, when British researchers in Antarctica were measuring the amount of ozone in the skies above. They found that ozone levels seemed lower in September, October, and November than at other times of the year. They compared their measurements to data collected every year since 1957 and found less ozone in the 1980s compared to earlier years.
At the same time, scientists at NASA's Goddard Space Flight Center were examining satellite measurements of unusually low ozone over Antarctica. After the British scientists published their results, the NASA scientists published the first images of an area of ozone depletion over Antarctica that was as large as the continent. The term "ozone hole" was soon coined to describe the seasonal decrease in the UV-blocking gas.
Satellite measurements of the ozone layer have been collected for nearly 35 years. Starting with the Total Ozone Mapping Spectrometer, NASA engineers and scientists have developed successive instruments onboard satellites, airplanes, the space shuttle, and the International Space Station to determine the amount of ozone in the air. For the past decade, the workhorse for ozone measurements has been NASA's Aura satellite and its Ozone Monitoring Instrument (OMI), which has extended and improved upon more than 30 years of observations. The recently launched Suomi National Polar-Orbiting Partnership satellite carries a successor to the OMI instrument that is continuing the record of observations.
How Will Our Vast Ozone Experiment Turn Out?
Piecing together clues from the laboratory and real-world observations, scientists eventually explained to politicians and chemical manufacturers why we needed to stop the production of ozone-depleting substances such as CFCs. The conversation led to an international agreement in 1987 known as the Montreal Protocol on Substances that Deplete the Ozone. Leaders with different political ideologies came together to create a model for global solutions to environmental problems. Many experts have called it the most successful treaty in the United Nations’ history.
The 1987 treaty set timelines for countries to reduce and eventually phase out the manufacture and sale of CFCs and other ozone-depleting chemicals. Motivated by the protocol, chemists, engineers, and manufacturers eliminated chlorine from most refrigerants and developed new chemicals that break down faster and lower in the atmosphere. The economic and environmental impact of the change from CFCs has been minimal so far.
While nations agreed to stop depleting ozone, nature will need time to catch up. CFCs are stable and long-lived compounds, and it will take a significant amount of time—estimated at 50–100 years—for the chemicals released decades ago to break down in the atmosphere. In fact, the worst global ozone losses and largest ozone holes occurred more than 15 years after the Montreal Protocol was signed.
The numbers tell the story of a changing atmosphere. In 1979, when scientists were just beginning to understand that ozone could be destroyed, the hole over Antarctica was 1.1 million square kilometers, with a minimum ozone concentration of 194 Dobson Units (DU). In 1987, the area of the hole reached 22.4 million square kilometers and ozone concentrations dropped to 109 DU. By 2006, the worst year for ozone depletion, the numbers were 29.6 million square kilometers and just 84 DU. By September 2012, the hole spanned 21.2 million square kilometers and measured 124 DU—the smallest hole since 2002.
As the Antarctic ozone hole has stabilized, there have been other promising signs. In the past decade, researchers found that the amount of ozone-depleting chemicals in both the lower and upper atmosphere reached a peak around the year 2000 and has been slowly declining. From the 1980s to the early 2000s, global levels of stratospheric ozone also dipped by 5% to 6%, but they have been rebounding slightly in the past few years.
The ozone hole is shown in purple above the Antarctic on Sept. 16 in the years 1979, 1987, 2006, and 2011. The Sept. 16 date was chosen because it is the International Day for the Preservation of the Ozone Layer. The maps were produced with data collected by satellite instruments, including the Ozone Monitoring Instrument on the Aura satellite. They show that the ozone hole kept increasing from 1979 to 2011, although to a lesser degree between 2006 and 2011.
Still, the experiment is not over. Ozone recovery is uneven, as the size of the hole still varies from year to year depending on weather conditions. In 2011, even though global CFCs were in decline, Arctic ozone fell to a historic low level due to unusual weather that allowed for greater than usual chemical ozone destruction. Although global warming and ozone depletion are caused by different gases, scientists have shown that a warming planet may change how the stratosphere—and, therefore, the ozone layer—works.
We seem to have avoided a future where there is no protective ozone. But our atmosphere is still vulnerable, and it is not clear whether the ozone layer of the future will look like the ozone layer we had in the past. It will take generations before we know how the experiment turned out.
Selected references
- Carlowicz, M.; Lindsey, R. Earth Observatory: The World We Avoided by Protecting the Ozone Layer, Earth Observatory, Goddard Space Flight Center, NASA, May 13, 2009:
http://earthobservatory.nasa.gov/Features/WorldWithoutOzone/page1.php [accessed Feb 2013]. - Wiscombe, W. Aura: A Mission Dedicated to the Health of the Earth's Atmosphere, Earth Observatory, Goddard Space Flight Center, NASA: http://earthobservatory.nasa.gov/Features/Aura/ [accessed Feb 2013].
- Allen, J. Chemicals in the Air: Latest Results from NASA’s Aura Satellite, ChemMatters, April 2008, 26 (2), pp 15–17.
Mike Carlowicz is a science writer from Massachusetts and the author of three books on the sun, moon, and space weather. He is the editor of the NASA Earth Observatory.
Keeping Tabs on Air Pollution from Space
Download video: MP4
Good vs. Bad Ozone
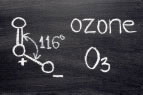
For living creatures on Earth, there is good ozone and bad ozone. Whether the molecule is helpful or harmful has nothing to do with the chemical makeup and everything to do with location. About 10% of the ozone in the atmosphere is found in the troposphere, the layer we live in; the rest is found in the stratosphere, about 10–50 kilometers (6–30 miles) above the Earth’s surface.
Good ozone is found in the stratosphere, far above the Earth’s surface. At that height, it absorbs and scatters ultraviolet (UV) radiation from the Sun, particularly the most dangerous UV-B and UV-C forms. Ozone is the planet’s natural sunscreen. Plants, animals, and all forms of life developed under a sky that shielded them from damaging and mutating radiation.
Ground-level ozone, on the other hand, is bad news. You've heard about it on hot summer days when your local weather forecaster says it's going to be a "bad air day." This ozone is often associated with smog (see article on p. 9) and forms from incomplete combustion—either the burning of forests and farmland or the incomplete burning of fossil fuels by vehicles, factories, and power plants. Sunlight cooks the polluting chemicals in the air, catalyzing chemical reactions that make ozone. Smog levels tend to be highest in the late afternoon.